
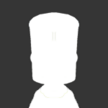
rdfox
Members-
Posts
760 -
Joined
-
Last visited
Content Type
Profiles
Forums
Developer Articles
KSP2 Release Notes
Bug Reports
Everything posted by rdfox
-
Gazelle and Sprint - fastest rockets ever?
rdfox replied to 1greywind's topic in Science & Spaceflight
Last-ditch endoatmospheric intercept of incoming ICBM and SLBM warheads; Sprint had a maximum range of 40km and a maximum altitude of 30km. These were not space launch boosters, but defensive weapons that, due to the high speed of their targets, had to react incredibly quickly. More information here: https://en.wikipedia.org/wiki/Sprint_%28missile%29 -
In 1967, NASA's Marshall Space Flight Center (or was it still the Manned Space Flight Center? I can never keep track of the name changes) in Huntsville was doing engineering studies for evolved Saturn launch vehicles for anticipated post-lunar programs. One proposal essentially took the first and second stages of FOUR Saturn Vs and clustered them together under a large payload bus; this was listed as theoretically being able to launch 1000 tons to LEO.
-
Gazelle and Sprint - fastest rockets ever?
rdfox replied to 1greywind's topic in Science & Spaceflight
Engineers who witnessed test launches of Sprint described it as less of a launch and more of an explosion. As a side note, the silo launcher was essentially a cannon that fired the missile into the air before it ignited, to further speed launch. -
something strange about the apollo program...
rdfox replied to JtPB's topic in Science & Spaceflight
Very belatedly (life has been kicking my butt recently!), but the time-remaining calls heard from CAPCOM during the landing phase on Apollo 11 referred to the length of time remaining in powered flight before the crew would have to either land or abort immediately, as the abort plan at that point used the descent engine to arrest the LM's downward velocity before the ascent engine fired, which means that they couldn't run the descent engine completely out of fuel before aborting. IIRC, post-mission analysis indicated that they had 20 seconds of fuel remaining before they would have hit the "must commit now" point when they landed. -
something strange about the apollo program...
rdfox replied to JtPB's topic in Science & Spaceflight
OK, for the record, the standard Apollo lunar parking orbit for the CSM was 60 nautical miles, circular. (Don't blame me for the units, they're what NASA used. That said, if you just use them as kilometer values, you end up with a fairly decent Munar situation!) The initial Lunar Orbit Insertion burn (LOI1) was done to a nominal orbit of Ap 160nmi, Pe 60nmi. (By way of reference, for Apollo 11, the post-LOI1 orbit was 160.9 by 60.9, referred to as a "damned accurate automatic burn" by the crew.) They then circularized the next time around. On the earlier missions, undocking would come in this 60nmi circular orbit, and, after a separation maneuver, the LM descent engine would be used for a Descent Orbit Insertion burn that would lower Pe to about 8 nmi (50,000 feet), some distance before the planned landing site. On later missions, the CSM would use its big engine (which had far, far more delta-V than was necessary for the mission) to enter this orbit before undocking from the LM, then would recircularize into parking orbit. (Returning to the standard parking orbit would eliminate the need to rewrite the ascent guidance software for both of the LM's computers--which was particularly problematic for the minimalist Abort Guidance Computer, which had minimal memory.) Either way, the LM would make an orbit or two before, shortly before Pe., using the descent engine for Powered Descent Initiation, the big braking burn that would gradually lower the Pe to zero--at which the computer would go to the next program, which automatically started to transition from orbital descent to vertical landing. If memory serves, this transition occurred at only about 3000 feet above the surface, and landing was vertical. From the start of PDI until the six-foot-long foot probes touched the surface, triggering the cockpit's contact light that told the crew to shut off the descent engine, the descent engine burned continuously, though the throttle varied throughout the burn, depending on what the requirements were. NASA decided against a "suicide burn" type landing (of the sort MechJeb does so well) partly out of conservatism, and partly to allow for situations like Apollo 11, where Armstrong saw that the automatic guidance was going to land them in a boulder field and had to take over manual control to fly past the boulders to a safe landing site. -
The reason for the extremely low SL Isp. is not due to any fundamental problem with nuclear-powered reaction motors, but purely because it was (understandably!) considered politically unacceptable to be using nuclear rocket engines in any application that doesn't see them put into orbit. Consider how much the people of the Azores, for example, would like having Saturn V first stages powered by nuclear engines being dropped into the Atlantic just a few hundred miles away... and then consider what happens to Titusville and Cocoa Beach if the rocket explodes at tower clear when it's being driven by nuclear engines--the NERVA design wouldn't generate significant fallout in an explosion *before* it was lit, but once you powered up the reactor, it became, essentially, a flying Chernobyl. Indeed, even if you didn't have an abort, the pad and mobile launcher would basically be single-use items with a nuclear first stage, due to the sheer level of contamination rendering it unsafe to have personnel attempt to refurbish them or otherwise work on them. (The only reason it was considered safe for manned flights was the bulk of propellant and tankage that were between the reactor and the crew, attenuating the radiation with distance and acting as shielding. IIRC, the S-N stage designed for use included RCS tankage to keep it oriented with the engine facing directly away from the spacecraft until it was a few kilometers away.) Nuclear reaction motors CAN be made to work well in an atmosphere; look up Project Pluto, a program to design a nuclear ramjet engine for an American cruise missile project of the 1950s. The only reason NERVA had nigh-nonexistent SL Isp. was because it was designed as a purely exoatmospheric engine, with the appropriate expansion ratio for that application.
-
As an idle note, during the late-60s development studies of "evolved" Saturn launch vehicles, Boeing/MSFC proposed a super-heavy-lifter option that would have put literally 1000 tons into LEO in a single throw. It consisted of four stretched, F-1A equipped S-ICs clustered as a first stage, with four stretched S-IIs clustered as a second stage, all attached to a common payload bus. That's right, they proposed essentially strapping four Saturn Vs side-by-side to build a super-heavy-lifter. Since there were no real applications for that kind of lifting capacity (I mean, really, other than launching an ISS-sized space station or a manned Mars mission in a single throw, what need IS there for the ability to put a WW2 destroyer into orbit?), this proposal never went beyond a paper study to determine the maximum feasible payload that they could get with evolved Saturn technology...
-
I wonder why Gs are higher during reentry than launch
rdfox replied to Pawelk198604's topic in Science & Spaceflight
For the record, in the real world, the acceleration loading on launch depends on the exact vehicle, while the G-loading on entry depends on the spacecraft AND the trajectory. For example, the Redstone, Atlas, and Titan boosters used in the Mercury and Gemini programs were converted ICBMs, designed to launch (relatively) tough nuclear warheads that didn't care much about high G-loadings. As a result, they made no effort to limit TWR in the later stages of ascent--the closest they came was the Atlas's "stage-and-a-half" staging where the two outboard booster engines (which made about 3/4 of the total launch thrust) were jettisoned two minutes into flight, but that was less for TWR control and more for a more efficient use of propellants. All three of them saw G-loadings exceeding 7g during the ascent; Titan II-Gemini actually did this twice, once near the end of the first stage burn, and once near the end of the second stage burn. I don't know much about the Saturn IB used for Apollo 7 and the Skylab and ASTP flights, at least in ascent G loads, but when it came to the Saturn V, as Mike Collins put it, "Saturn is a gentleman," with maximum G loadings of about 4.5g during ascent, thanks to the planned early shutdown of the center engine in the first and second stages--which was done solely for TWR control without the added complication of a throttle. Shuttle maintained a 3g maximum through throttles on the SSMEs and careful shaping of the SRB propellant castings to control thrust levels. As for entry G loadings, KSP re-entries are currently ballistic, as the aerodynamic model doesn't simulate spacecraft body lift, only wing lift. In reality, the only manned spacecraft designed for pure ballistic re-entries were the Mercury and Vostok spacecraft. While I don't have any access to the Vostok's entry G-loading data, I do know that Mercury's G-loading soared on entry, particularly on the two suborbital flights (which, by nature, had a steeper entry angle than the orbital flights). If you listen to the cockpit tapes of Al Shepard's Mercury flight, you'll hear him reading out G-loadings during the entry. "Three... five... seven... *grunt* nine... ELEVEN... I'm OK... OK... OK..." After the parachute had deployed, he reported to the ground a peak G-loading of about 11.5g during the entry. All other capsules, as mentioned above, had/have their center of mass *not* along the vehicle centerline, so that their aerodynamically neutrally stable attitude is tilted slightly, to generate some lift and reduce the entry G-loading. For return from low Earth orbit on a normal lifting entry trajectory, there's a handy rule of thumb--the peak entry G-loading is equal to the reciprocal of the spacecraft's lift-to-drag ratio. For example, the Gemini capsule had a lift-to-drag ratio of 1:4, which meant that the peak entry G-loading in it was about 4g. (I'm not sure this held true for the Space Shuttle, but it flew an extremely shallow entry profile designed to reduce peak thermal loading at the cost of a much longer thermal soak, to suit the reusable thermal protection system employed; the ablative TPS used on capsule-type spacecraft can handle a much higher peak thermal load, but is more limited in the length of the acceptable exposure.) However, attempting to use a "normal" shallow entry profile on Apollo wasn't an option, except on 7, 9, and the Skylab and ASTP missions. Coming back from the Moon, there was no braking burn into Earth orbit before entry; they flew a direct entry trajectory that saw them plummet straight into the atmosphere to land. Using that shallow of a profile would have resulted in the spacecraft "skipping" off the atmosphere and off into a high Earth orbit that would not return before the command module's consumables were used up and the crew was dead (some "skip" profiles would even see them end up in an orbit that would never re-enter the atmosphere!). As a result, a much steeper profile had to be used, and the L:D rule of thumb didn't work any more. A normal Apollo lunar re-entry would hit about 7.5g, and if the trajectory was a bit off but still within survivable limits, that could soar as high as 14g. (As a result, all Apollo Command Module Pilots had to be trained to fly the entire entry--solo, in case the Lunar Module crashed or couldn't launch from the surface--at 14g in a centrifuge, which, according to Collins, was "no fun at all.") (As a side note, there *was* a contingency plan for Apollo, in case the designated landing zone was forecast to be in a typhoon at landing, to deliberately use a trajectory that was shallow enough to create a small atmospheric "skip" before the terminal entry and shift the landing point a few hundred miles into hopefully better weather. Since this was never tested unmanned, the crews were understandably a bit apprehensive about actually using it...) -
From my amateur analysis, and listening to last night's press conference: At about T+6 seconds, one of the first-stage engines, for whatever reason, suffered a Rapid Unplanned Disassembly, resulting in the first explosion and, with total loss of half the rocket's thrust, the start of a "pad fallback" accident. Following this, at about T+15 seconds, the Range Safety Officer sent the ARM command to the Flight Termination Package, which also sent a shutdown command to the remaining engine; following protocol, the RSO then waited until about T+20 seconds to send the DESTRUCT command, venting the propellant tanks and blowing out the side of the second stage to ensure that A) the liquid propellant would be somewhat dispersed at ignition instead of all being concentrated directly on the launch pad, and that the solid-fueled second stage would not "cook off" and ignite in a propulsive manner after impact. This is the bigger explosion you see, and the chunks of flaming debris that shower outward are parts of the second stage, broken apart by the combination of the FTS linear shaped charges and the blast from the first stage deflagration. That first-stage blast is also why, despite the rocket having started to fall back onto the pad, the solid fuel chunks also head *upwards* initially. Accidents are never good, but both NASA and Orbital have to be feeling very lucky that this one happened at such low altitude; while it did do a lot of damage to the pad (although, in the press conference, they mentioned that instrumentation showed that the various tanks at the pad were still maintaining pressure (which means that the damage is superficial and relatively minor!), a low-altitude failure means that the debris field is much smaller and easier to recover (for analysis) and clean up (for environmental remediation)--plus, on a purely economic note, it means a smaller security cordon/perimeter that needs to be maintained, and thus a smaller security *force* that needs to be maintained, which, of course, means lower costs in keeping the scene secure. This one happened low enough that damage appears to be confined solely to WFF/MARS LP-0A, and the remaining fires should have burned themselves out by this time, allowing the investigators to head in and start documenting and collecting the debris. The classified crypto gear mentioned as being on board is almost certainly simply communications equipment for secure communications with the ground from the USOS. Those sorts of secure radios are all over, but are all classified simply to protect the details of the encryption scheme they use. Hardly a military "toy," it's just a way to talk to the ground about (for example) how Bill's motion sickness is slowly improving and he only puked twice today, *without* the public listening in. US manned spacecraft have all had those since the later part of the Apollo era, after the astronauts on Apollo 8 had to use a rather roundabout secondary communications method (normally used for engineering data only) to privately discuss Frank Borman's motion sickness with the ground. (And no, it's not about trying to keep secrets; it's about the fact that sometimes, they have to discuss personal matters with the ground that various privacy acts say the government cannot allow to be broadcast to the world...) The ISS program is very conservative about the chances of a failed supply mission; they generally keep enough consumables on board the station for 4-6 months to ensure that a failed supply mission will not be critical. The current stockpile (with both a Dragon and a Progress having just left the station in the past week) is at the high end, and even if the new Progress had failed and the December Dragon launch was scrubbed, the crew could stay aboard into March or April without any further resupplies. The biggest effect this will have on ISS operations is a reduction in the experimental work that the crew will do for a few months, pending the next Dragon flight. Of course, this sort of thing WOULD have to happen on the very day I "cut the cable" on my TV...
-
Question about cost breakdown of a real Rocket, E.g. Falcon 9
rdfox replied to Raukk's topic in Science & Spaceflight
Consumables cost is very easy to calculate. Market prices for the various sorts of rocket propellants are easily found through Google, and the capacities for said consumables are public information. Multiply the market price per unit volume (or unit mass, as appropriate) by the booster's capacity, and you have the price for any given consumable for each launch. Add those up, and you have the consumables cost of the launch. For example, if one was to launch a Saturn V today: The S-IC first stage carried 810,700 liters of RP-1 fuel and 1,311,100 liters of liquid oxygen oxidizer. (All capacities per NASA's official technical history of the Saturn boosters, "Stages to Saturn.") Other than the eight solid-fuel separation motors (which I cannot find cost data for), it used no other consumables, as it steered entirely via engine gimbals, and the hydraulic system for gimballing the engines used RP-1 as its actuating fluid. This works out to 214,164.28 gallons of RP-1 kerosene and 1647.57 short tons of LOX. (Conversions courtesy of aqua-calc.com, and you'll see the reason for the odd selection of units momentarily. Aqua-Calc doesn't cover liquid hydrogen or hypergolics, so I used conversion tables available at http://www.uigi.com/h2_conv.html for LH2, and density data on Wikipedia for others) According to a public listing from the Defense Logistics Agency (http://www.energy.dla.mil/customers/standard_prices/Documents/Aerospace%20Energy%20Standard%20Prices/Prices%20for%20FY%202008.pdf ), in US government Fiscal Year 2008, RP-1 cost $3.50 per gallon, and LOX (at Kennedy Space Center, where there was a production plant on-site not requiring transport) cost $66 per ton. This means a cost of $749,574.98 for the RP-1, and $108,739.62 for the LOX, or a total first-stage consumables cost of $858,314.60. Continuing up through the Apollo/Saturn V stack: S-II: 331,000L LOX, 1,000,000L liquid hydrogen. This works out to $27,452.45 for LOX and $468,164.79 for LH2. S-IVB: 253,200L LH2, 92,350L LOX, 95L nitrogen tetroxide, 114L monomethylhydrazine. $118,539.33 LH2, $7,659.32 LOX, $6,644.49 N2O4, $23,336.97 MMH. Lunar module: 3800L N2O4, 4500L Aerozine-50 hydrazine. $265,779.60 N2O4, $953,306.51 Aerozine-50 Command and Service Modules: 9727L N2O4, 8000L Aerozine-50, 379L MMH. $680,325.84 N2O4, $1,694,767.12 Aerozine-50, $77,585.19 MMH. I couldn't find numbers on the total capacity of breathing oxygen in the LM and CSM, so we'll count propellants only. This adds up to $1,510,111.95 in consumables to launch a Saturn V in 2008; if we add the Apollo spacecraft as a payload, it increases to $5,181,876.21. By comparison, Wikipedia lists the 1969 cost of a Saturn V, including launch, at $185 million, which adjusts for inflation to $1.094 billion. Thus, the consumables cost of launching a Saturn V would be about 0.1% of the total launch cost. Moral of the story: propellants are cheap. It's everything ELSE that's expensive. -
Extremely geeky video about making the Apollo Guidance Computer
rdfox replied to Beowolf's topic in Science & Spaceflight
There's a reason that the astronauts had quick-reference cards for what the Verb and Noun numbers were. And why, when they weren't doing routine procedures that were written out in the checklists, the exact sequence of keystrokes required were worked out on the ground, then transmitted up to the crew to transcribe, then the crew would read them back to the ground to verify that they had transcribed the correct sequence, and then corrections would be sent up, then the crew would read back the corrected sequence, etc. etc., until it was confirmed that they had the correct sequence of keystrokes written down. Yes, it was an awkward procedure that was hated by the crews. Yes, there were originally plans to include a teletype on the Apollo spacecraft for sending such strings of numbers and keystrokes without the chance of transcription error by the crew (this was deleted for weight reasons). Yes, this is why the Shuttle originally flew with a teletype, and why it was later refitted with a fax machine (same purpose, but broader capabilities for less weight), and why the ISS has a printer on board to print out emails from Mission Control. And yes, this IS the same procedure used for instrument flight clearances to this very day in the aviation world... -
There is a point where the term "diminishing returns" begins to apply to recovering booster stages. I think that attempting to recover F9H core stages would be well beyond that. If SpaceX wants to reduce cost of the F9H by recovering engines from the core stage, I think they should look towards the past--specifically, the Atlas booster, and the S-ID first stage for evolved Saturn Vs proposed in the late 60s. Both used a "stage and a half" design where the outboard engines were mounted on a "collar" that could be jettisoned once they were no longer needed. While the Atlas's booster engines were expendable, the S-ID design was intended to not only increase payload, but to also reduce cost by having the four outboard engines recovered and refurbished for reuse on a future launch. The inboard engine on the S-ID would be a sustainer, and would not be recovered, but by recovering four out of five first-stage engines, the bulk of the stage cost could be recovered, compared to the S-IC. The new F9 design, with the circular ring of outboard engines as opposed to the square nine-pack design used on the early boosters, would be ideal for a similar "stage-and-a-half" setup; it could even be configured as a dual-collar design, such that only four engines are jettisoned at a time, allowing even earlier reductions in thrust (and making recovery of all the engines simpler due to having less weight to land safely)...
-
Retroprolpulsive landings vs parachutes
rdfox replied to FishInferno's topic in Science & Spaceflight
Parachute-only landing is *not* limited to splashdowns. For unmanned single-use spacecraft, a "heavy" landing (typical parachute descent speeds at impact are <10 m/s, equivalent to roughly 20 mph/32 kph) isn't a big issue, as the hardware can take more of a beating than squishy human bodies. For manned spacecraft, it's more of an issue, but not a critical one--all of NASA's capsule-type spacecraft were designed to make land ("contingency") landings without significantly injuring the crew. (They would have bumps and bruises, but no open wounds, broken bones, or concussion from the impact.) This was done because, under certain wind conditions, a launch abort off the pad or in the first twenty to thirty seconds of ascent could, theoretically, see the spacecraft blown back onto land by the wind, in addition (in Apollo) to providing a "wherever they come down" landing capability in the event of a post-TLI abort on a lunar mission requiring a turnaround rather than a lunar swing-by a la Apollo 13. The method used was by using crew couches designed for shock absorption, mounted on struts specifically designed, should there be excess accelerative loadings on impact, to crush and thus provide cushioning to it. (The struts were actually quite similar to the crushable shock absorber structure in the LM landing gear.) A water landing was the preferred option, by far, but a land landing would be survivable, even factoring in the need to exit the spacecraft and survive until the SAR forces found you. The one flown exception to this was Apollo 7, where the Block 2 Apollo CM used had to be flown with the Block 1 CM's crew couches, as the Block 2 couches wouldn't be ready until Apollo 8. Testing after the AS-204 fire found that the Block 1 couches were marginal, at best, in a "contingency" landing; as a result, Apollo 7 was the only Apollo mission to have a special mission rule that put more restrictive limitations on the winds at launch, to ensure that an abort would see the spacecraft land in the ocean. NASA has acknowledged that they did NOT want to deliberately target a land landing with a pure parachute design, at least for a round parachute design, because it was likely to beat up the astronauts and they couldn't *guarantee* that there wouldn't be serious injuries. (The initial impact would not be a problem; however, if the spacecraft tumbled down a slope, the crew might well be injured.) This is why, during the period when Orion was expected to eventually transfer to landing at Edwards AFB instead of the ocean (to reduce refurbishment requirements for reuse of the capsule), the plan was to carry a Soyuz-type retro-rocket to cushion the landing. Of course, the Gemini spacecraft was originally planned to be the great exception, using a Rogallo parafoil wing to glide to a landing on a runway at Edwards, requiring no impact cushioning at all due to the use of lift to touch down as gently as the Space Shuttle eventually did. (The parafoil was only dropped for time reasons; development had hit snags, and a circular parachute was substituted to keep Gemini's support of the lunar program on time.) Given that such parafoils are now widely used by skydivers, and the US military is testing combining them with GPS guidance systems to allow precision airdrops of supplies and equipment, use of a parafoil for a runway landing may end up being the preferred primary option in the future, with a circular parachute system as a contingency option... -
As a side note, one of the wonderful (for some value of "wonderful") things about treaties is that signatories *can* choose to withdraw from them at any time. What's more, they can be modified, if necessary, so long as all signatories agree to do so. And the only *real* force that they hold is that they're an agreement not to do something--the only penalty for abrogating one is that it frees the other parties to do likewise. A fine example of these is the 1936 London Naval Arms Limitations Treaty, which set a limit on the size of new battleships to attempt to maintain the "curbing" of the naval arms race that the 1921 Washington and 1931 London NALTs had started. New battleships were, under the treaty, to be limited to a size of 35,000 tons; the treaty also included an "escalator" clause which, should the signatories learn of a non-party to the agreement--i.e., Japan--building battleships that exceeded the Treaty limits in size, would allow this size cap to be raised. In 1937, following people learning of the Yamato-class battleships under construction, the escalator clause was invoked, raising the limit to 45,000 tons. However, it appeared that even the raised limit would not be sufficient for the designs that the US was developing for construction starting in 1938, and various "paper" measures were taken to reduce the theoretical weight of the ships "in fighting condition"--setting a nominal ammunition load of two-thirds the actual maximum capacity, similarly limiting the nominal consumables carried versus actual capacity, etc.--to get a "standard" displacement close enough to the limit--within 100 tons or so--that nobody would complain. However, with the outbreak of war in 1939, all parties tacitly agreed to suspend the Treaty, which allowed the Iowa class battleships to be outfitted with equipment that took them far beyond the Treaty limits; even going by the "standard" displacement calculation of the Treaty, about the lowest you could get the ships as actually built was 51,000 tons. Ironically, postwar, the French stated that they considered the Iowas and the Midway-class carriers (which were almost double the 23,000 ton limit for aircraft carriers) to be abrogations of the treaty, and it has been considered discarded ever since.) In the event of a planet-threatening impact event, as mentioned above, the world community would likely simply ignore the OST and clean up the legal mess afterwards. (As a parallel, when a madman stole an M60 tank from a National Guard armory and went on a rampage with it in San Diego in 1993, the local Marine Corps airbase started preparing a couple of attack helicopters with anti-tank missiles to destroy it; had the driver not disabled his own tank by getting it high-centered on a concrete highway center divider, allowing police to storm the tank, the helicopters would have been sent out, despite a longstanding US federal law called "posse comitatus" that flat-out bans the use of the Federal military for any form of civilian law enforcement--the feeling was that the exigency of someone driving a 50-ton tank through civilian neighborhoods, crushing vehicles, attempting to knock down overpasses, and then driving on the freeway was too critical to allow him to continue to pose a threat, that he had to be stopped by any means necessary, and that they'd find some way to clean up the legal mess once they didn't, y'know, have a friggin' TANK trying to run over rush hour traffic.) However, in the event of a lesser threat--say, a regional one--a spacefaring nation that was going to be threatened could choose to simply act unilaterally, ignoring the OST, and choose one of four options when doing so: A) Argue that the OST does not cover Peaceful Nuclear Explosions, and that attempting to deflect an asteroid from impacting Earth would qualify as PNEs. (There is some precedent for this; the Limited Test Ban Treaty of 1962 prohibited nuclear weapons tests in the atmosphere, underwater, and in space, limiting them to deep underground shots only; however, a number of shallow-underground tests that were deliberately intended to breach the surface were conducted as part of American and Soviet programs to attempt to find peaceful uses for nuclear weapons, both in the US Project Plowshare, and the Soviet project of Nuclear Explosions for the National Economy. Indeed, the Soviets even made some operational use of PNEs to redirect rivers and create reservoirs.) It would be a questionable interpretation of the treaty language, but it could be argued. The nation could initiate negotiations to amend the OST to specifically allow missions to deflect asteroids away from Earth. While it might be seen as a violation to carry out a mission to do so while negotiating a change to the treaty to permit carrying it out, it would be politically very dicey to push TOO hard on that issue, if the nation in question is trying to negotiate in good faith. C) The nation could simply elect to withdraw from the OST and no longer be held by the bounds of it. This might result in sanctions against the nation, but again, in this situation, I suspect that the response from the international community would be muted, since they would be withdrawing for reasons that are clearly not warlike. D) In what I consider to be the preferred option, the nation could just go ahead and do it and not take any action regarding the OST; if anyone complained, they could politic against any action against them--which would likely be unpopular anyway, given the nature of the treaty violation. Russia might gnash its teeth over an American use of nuclear weapons to deflect an asteroid that threatens the United States, for example, but they would likely find little support, internationally, for bringing any sort of sanctions against the US for doing so. These options also would apply to the Limited Test Ban Treaty and the Comprehensive Test Ban Treaty, both of which prohibit setting off nuclear weapons in space. Of course, this all assumes the asteroid is found to be threatening with relatively little time before impact and the "brute force" approach is the only deflection option. If it is found early enough, far less-violent means of deflection would be sufficient, since a net delta-V of 1-2m/s would result in an immense change in trajectory after ten years! Additionally, it's unlikely that even a lower-rated impact threat on the Torino scale would see anything but a full international response, because the imprecision in tracking the object when it's still early enough to deflect it makes it impossible to set the exact GMT time of a potential impact, or its location on the planetary disc, or its exact entry trajectory. These make it impossible to make early predictions as to exactly what nations would be affected, and thus mean that it would be impossible to say, early enough to allow deflection, that a T3-T5 object would threaten, say, Europe, but not Asia or the Americas, and thus would make it difficult to have that sort of unilateral opposition to a deflection mission on the grounds that if it hits INSERT OTHER NATION HERE, it's just too bad...
-
Solar panels do NOT deploy through fairings; I've had cases where I was using a fairing and it somehow got "hung up" on something on jettison and was sticking to the vehicle; the only way I could kick it off of the spacecraft during the boost phase was to deploy the solar array underneath it to push it out and away.
-
Looks like a routine belly landing. Most likely, if you just jack her up, lower the landing gear, and replace the prop and engine (which will need a full teardown and inspection if not a full rebuild due to the torque loads imposed on it), she'll be airworthy for at least a "hospital" ferry flight immediately. Propeller-driven warplanes, with those big piston engines, were *tough* birds (so were propliners), because of the vibration environment that they lived in. (On the prototype 707, there were a large number of gauges that didn't work on the early flights without the pilot tapping them any time he wanted to read them, because they were designed for propliners and had lots of resistance in them so that they'd read steady in all that vibration... and on the 707, there was no vibration, so they just tended to stick in place!) Put it to you this way--I've personally ridden on a B-17 that suffered damage that, by all rights, should have written it off TWICE. The first time was in 1953, when it was used as a target in military effects (i.e., damage) studies at three nuclear tests in Nevada. After being repaired in 1965 (cannibalizing parts from another essentially-destroyed B-17 that was used in the same tests), it was restored to service for use as a water bomber for fighting forest fires... pretty much just in time for the US Forest Service to ban the type from water bomber service; it was then converted back to regular bomber configuration and became an airshow warbird, until, in 1986, a botched landing saw it go through the fence at the end of the runway, tear off its landing gear on a drainage ditch, and lose an engine and the outboard part of the right wing to hitting a telephone pole. While this would have been considered fatal damage and resulted in a trip to the scrapper just ten years before, the owner decided to repair her, and by 1990, the bird was flying again and remains on annual tours around the US to this day. So yeah, most likely, that Sea Fury will be flying again pretty soon.
-
There's another "reference" Kerbal name out there, but this one is tied to another specific Kerbal model, not just a generic one. That would be Bobak Kerman, seen gasping (and later high-fiving Gene) in this particular KSP promotional video: Asteroid Redirect Mission promo video As made clear by the shirt and hairstyle, he is a reference to Bobak Ferdowsi, famous to space fans as JPL's "Mohawk Guy" from the Curiosity landing.
-
When I design my ships, I tend to plan for about 110% of the delta-V required for the actual mission profile. I then get as close as I can to that number (without going below 110%) using the standard parts; this usually has me in the range of 115%, though on some of my high-Isp, low-thrust transfer stages, I've had it be a case of, "well, I can either have 90% of the required delta-V, or 200% of it, and NOTHING in-between... guess I'll go 200%."
-
Why were the SSMEs at risk of damage if run dry?
rdfox replied to photogineer's topic in Science & Spaceflight
Another issue is that, in the event of an oxygen-rich shutdown situation (i.e., the fuel supply runs out first), the cold LH2 fuel being pumped through the cooling passages in the engine nozzles will run out before the combustion ends, resulting in severe burning of the engine nozzles themselves due to lack of cooling. (This was actually specifically called out in the Rogers Commission report on the Challenger disaster as further evidence that the SSMEs were not the point of failure, as all three showed the sort of nozzle burns that were to be expected from oxygen-rich shutdowns. That came due to the LOX tank in the external tank being the upper tank, with the longer line supplying it to the engines, so when the ET disintegrated, the LOX supply in the plumbing lasted longer than the LH2 supply.) If you ablate the nozzles enough, you'll get a burn-through structural failure, which is a Bad Thing (it can result in Rapid Unplanned Disassembly) even in an expendable engine. This is why just about all rocket designs have included low-level sensors in both the fuel and oxidizer tanks which would, when a certain number of them simultaneously indicated a low-level condition, initiate a controlled shutdown of the engines; it meant that shutdown would not come due to starvation of one propellant or the other and result in a loss of regenerative cooling of the nozzle and potential burn-through. With the SSMEs designed to fly 20-30 times, this clearly becomes even more important than it is in a simple pressure-fed expendable engine, as damage suffered in one flight may result in failure on a later flight; between that and the various turbopump issues (cavitation when pressure gets too low, loss of lubrication from loss of the pumped fluid, and overspeeding due to loss of resistance of the pumped fluid), a controlled shutdown with significant residual propellant remaining was the safest option. (Interesting note: it's not just the turbopumps on rockets that use the fluid that they pump to restrict their speed and provide lubrication. The in-tank electric fuel pumps on modern cars are designed to use the gasoline they pump into the fuel lines to both keep the pump from overheating, and to lubricate it so that the bearings don't fail. Thus, it's really a bad idea to let your car run out of gas, because it may do some damage to the fuel pump... and refilling the fuel lines when you try restarting it after getting fuel in the tank almost certainly will. It won't *break* it, not the first time, but it can make it weaker, and more likely to eventually fail.) -
I really shouldn't suggest this (because I sure as hell can't do it!), but how about a mission to put a communications satellite into kerbinstationary orbit... at a specific longitude? (After all, commsats are useless if you put them into an orbit where they're below the horizon for the relay stations they're supposed to connect!) Maybe with a tolerance band ("100 degrees west longitude +/- 5 degrees") to allow for the inherent imprecision of our flying and our simulation, but this would probably be an accurate simulation of the single most profitable, and largest, sector of the contract spaceflight industry...
-
Actually, given the requirement, I suspect that Boeing would prefer to build a winged vehicle, since this is merely a first stage. In fact, I suspect that said winged vehicle will look suspiciously like it shares its wings and engines with the 747-8, but with a body designed specifically for the mission to allow the booster to be dropped from it without the violent negative-gee maneuver that would be needed to separate from it if you were to use Shuttle-style carriage on top of the existing 747 fuselage. (When the 747 was used for Enterprise's drop flights in the Approach and Landing Test series, even with the lift of the Shuttle's wings, the 747 had to make a zero-gee pushover to separate from Enterprise, due to the Shuttle's poor glide ratio; with an essentially wingless upper-stage booster, you'd need to make it even more aggressive, and negative gees are hard on both pilots and airframes.) Really, if you want a fully reusable first stage, that's the simplest way to do it--use a large airplane for the "initial loft" up to about 15-17km and 250-300 m/s horizontal velocity, then drop the rest of the booster from it and have it fire once clear; the airplane then flies back to base. This has a long and proud tradition, from the early X-planes (X-1 and variants, X-2, X-15, from B-29s, B-50s, and B-52s) to today's Pegasus/Pegasus XL (from B-52s and L1011s) and Rutan's SpaceShipOne and SpaceShipTwo (from the White Knight series motherships). Indeed, the USAF even tested the capability of launching ICBMs that way once in the mid-1970s, in a capability demonstration where a Minuteman ICBM was rolled out of the rear cargo ramp of a C-141 in flight and then fired to fly a normal mission. (The idea there was that it would allow the Air Force to compete with the Navy for having a "survivable" rapid-response deterrent force, in that transports flying around the US with ICBMs on board, ready for in-flight launch, couldn't be pre-targeted by Soviet missiles, much like the Navy's ballistic missile submarines at sea...)
-
Technically, tonsillitis is any form of inflammation of the tonsils. And while it used to be standard procedure to just automatically do a tonsillectomy any time someone had it, at least in the US, by the late 1980s, that was no longer the case--when I had it back then, the doctor explained that it was now recognized that the tonsils had an important purpose (they help catch things falling down the esophagus that could cause infections) and that tonsillitis was actually them doing their job properly by keeping an infection from getting further into the gastrointestinal tract. So, instead of a tonsillectomy, he prescribed bedrest, lots of fluids (which were about all I could swallow at the time, anyway), and antibiotics to help fight the infection. Still have my tonsils today, as a result...
-
N-1's hidden in a top secret siberian hanger
rdfox replied to xenomorph555's topic in Science & Spaceflight
The N1 *couldn't* be transported to a secret warehouse in Siberia and stored on site. One of the main sources of the problems that plagued it during the test flights was related to its sheer size and the Soviet infrastructure. Saturn V wasn't a problem for the US, because even though the first two stages were 10m in diameter (yes, that's accurate--the Apollo hardware is all friggin' HUGE), NASA and its contractors had one major advantage--the launch site was located just off the Atlantic Ocean, making sea-based transport of the outsized stages entirely feasible. Thus, the S-II (second stage) production facility was built in Seal Beach, California, with direct ocean access, while the S-IC (first stage) production facility was the NASA-owned Michoud Assembly Facility at the Stennis Space Center in Mississippi, just off the Mississippi River, with test stands located not far away, also with access to the Mississippi River. Even the first two flight articles, built by the engineers at Huntsville and tested in test stands there, could be transported by water, because of a roundabout series of navigable rivers that provided access to the Gulf of Mexico. Thus, barge shipment was the preferred method, (The S-IVB third stage was also built along the California coast, though without direct water access--shipping it required it to be trucked through town to the docks to be loaded on the barge. However, it was also small enough in diameter that the Super Guppy aircraft could carry it, and since the contractor had an airfield right on the property next to the factory, air transport became its preferred mode.) Every S-IC and S-II stage was shipped from the factory to the test stand by barge (through the Panama Canal, in the case of the S-II), then erected on a test stand and given a test firing of at least its full mission operating cycle, all the little bugs fixed, and then test-fired again to prove that there were no more bugs in the system, then shipped to the Cape, by barge, to be assembled into a flight-ready vehicle and flown. By contrast, the Soviet launch site, while as low-latitude as they could get it (for maximum boost from Earth's rotation), was also completely land-locked, hundreds of miles from any navigable waterway. Everything had to be able to be shipped in by rail, truck, or airplane--and this meant that the N-1 couldn't have its lower stages assembled where they were fabricated, or be test-fired before flight--instead, they were fabricated in sections, shipped by rail to Baikonur, and then assembled on site. The Soviet government refused to build full-scale test stands at Baikonur, so these complex rockets, assembled in relatively primitive conditions by relatively unskilled laborers, were never test-fired before launch. In a ground test, if there's a problem with the stage, you can shut it down, safe the vehicle, diagnose and fix the problem, and then try again. However, if the first time the rocket is fired is in *flight*, then you're pretty much screwed, since shutting down will still result in the destruction of the vehicle. (Yes, theoretically, a "Flight Readiness Firing" test of the N-1's first stage on the launch pad could have been done. This would have greatly slowed the processing rate, due to the need to do it for each booster, could have severely damaged the pad and upper stages, and, at best, would have shaken the bugs out of the first stage only.) Since the Soviets couldn't transport the N-1 stages in one piece, it makes transporting the "extra" ones to some mythical Siberian warehouse infeasible--what are you going to do, dismantle them into their original component parts (including "un-welding" the welded joints in the tanks), load them onto trains, ship them out there, and then reassemble them on site for storage?--what they did made the most sense--partial disassembly to retrieve the most valuable parts (electronics and engines), and then simply scrapping the rest, since what would be left would essentially be structure, tankage, and plumbing--which are cheap (by comparison). -
If you could ask Sergei a question...
rdfox replied to xenomorph555's topic in Science & Spaceflight
Actually, one of the first things I'd ask him is what he thinks of the US's current workhorse expendable booster being powered by the RD-180... After that, I'd ask about some of the things that still aren't clear about the Soviet program, like whether the N-1's first stage design was due to an inability to solve the combustion instability problems that plagued very large single-barrel engines (the Saturn V's F-1 engines blew up regularly on the test stand in the early part of development), limited test stand capacity for large engines, or an early attempt at creating the effect of a toroidal aerospike engine with the air venting through between the outer ring and central core of engines. It'd be nice to find out exactly *why*, in that example, they decided to go with thirty little engines instead of a smaller number of larger engines. Beyond that, I'd also want to get his opinions on various rocket designs, ranging from real-world ones like the SpaceX Falcon series, to studied-but-never-built ones like the Saturn V derivatives proposed in the late 60s (including the S-ID "stage-and-a-half" version of the first stage, which would work like the Atlas booster and jettison the outboard engines once they were no longer strictly necessary, though unlike Atlas, the proposal was that this would allow recovery and reuse of the outboard engines), to even possible ways that he could have further developed the N-1 for the future, had it succeeded. (Again, my first thought on the N-1 is making it a stage-and-a-half first stage design, jettisoning either the outer ring of engines, or, if they were intended to act as a toroidal aerospike, jettisoning the *inner* core engines...) -
Whoops! Well, while my specific numbers won't work, the same basic concept should still apply--don't bother trying to get a circular orbit, just try to fly a gravity turn to a comfortably high apoapsis, then use the remaining solid motors prograde at that apoapsis to insert into an elliptical orbit like done with the real one... I'd expect that would still get you into SOME sort of orbit, anyway.